WHY DO WE WANT EVEN HEATING AND WHAT CAN WE DO ABOUT IT?
The job of the stovetop cookware is to smooth out the uneven heat coming from the burner underneath so that the cooking surface of the cookware is the same temperature. If you have too much of an imbalance in temperatures, you wind up with hot spots that can undermine your dish and your health by leaving some food overcooked (or even charred and carcinogenic) and some food undercooked. For instance, if you put a poor thermal conductor on a burner, the heat from the burner will keep accumulating near the areas where the flames touch the cookware because the cookware can’t spread the heat across the entire pan’s base fast enough.
HEAT TRANSFER
There are three ways to move heat from one place to another: convection, radiation, and conduction.
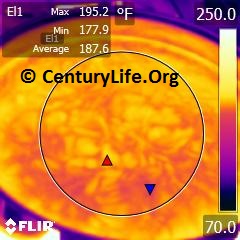
Convection is transfer of heat from one place to another via liquid/gas movement. In the cooking context this happens when a fluid is heated up from a single direction, such as boiling water: some parts of the fluid will get hotter faster, but they will also move away from the heat source due to density differences, allowing colder fluid to heat up, and so on until all of the fluid is heated up to about the same temperature. Excess heat simply vaporizes liquid water into water vapor.
In cooking, anytime you have something very liquid (that is, thin liquid such as watery soup rather than thick stews), you will have even cooking due to convection, and consequently, it almost does not matter what the cookware is made out of–you will get fairly even heating. As an example, the bottom of a boiling pot of water will be barely any hotter than boiling water–for any decent cookware, about 222F instead of 212F (105C instead of 100C). Similarly, many ovens will circulate air through forced air convection (with a fan or pump) and thus evenly heat anything inside of the oven. Ovens make even the worst cookware perform like champions when it comes to even heating, because the entire cookware is being heated all at once and all at the same temperature.
Radiation is transfer of heat through emission and absorption of radiation. Electromagnetic radiation can travel through empty vacuum or transparent medium like air and then be absorbed by something which gets excited and hotter as a result. This is the way that the sun heats the earth through space, for instance. It is also how a microwave heats up food.
Conduction is the direct transfer of heat from materials in physical contact with each other. You can think of it as molecules bouncing off each other and doing the microscopic equivalent of rubbing hands together to generate friction heat. Since solids typically have the highest density of molecules per unit volume, we tend to think of conduction as a solid-to-solid heat transfer, but liquids and gases also conduct heat. Electric coil/radiant/halogen stovetops rely on heat transfer from cooktop surface to cookware. Gas stovetops do not rely on only conduction; they rely on hot gases to swirl up and around cookware via kitchen air convection currents. That is, heat rises from a burner tip and hits the bottom of your cookware, then swirls up and over the bottom of the cookware into the air in your kitchen. Electric induction excites the molecules in your cookware directly.
Regardless of cooktop type, once any part of a solid cooking vessel is heated higher than ambient (room) temperature, the cooking vessel itself will conduct heat to other parts of the cooking vessel. When it comes to stovetop cooking of non-liquidy food, conductivity of the cookware is what makes it heat evenly or unevenly, so it is what we’ll be spending most of our time discussing here. (This even applies to radiation-based heating: once the exterior of the cookware is heated up, it is the thermally conductive layer of the cookware that makes it spread heat evenly or not.)
If you want a longer explanation of heat transfer, see this Wikipedia article.
THERMAL CONDUCTIVITY
Imagine that heat is water, and that metals are like water pipes of the same diameter.
Silver is the most thermally conductive metal. It would be like a completely unclogged water pipe, where a certain amount of water can flow through it per second.
Every other metal would be like a clogged pipe. Copper is almost as thermally conductive as silver, so copper would be like a barely-clogged pipe. Aluminum would be like a half-clogged pipe. Cast iron would be mostly-clogged, and so on and so forth. (Insulators such as air would be like almost-completely-clogged pipes.)
You can make up for partially-clogged pipes by using more of them. For instance, two half-clogged pipes will give you the same throughput as one unclogged pipe. They take up more space, but maybe that does not matter when the pipes are being measured in millimeters rather than inches. We’ll discuss that more, later in this article.
You can also make up for partially-clogged pipes by easing down the water pressure. If you overstuff water pipes, the water pressure will build up until the pipe material breaks, and the water pipe bursts. In the cookware context, if you heat a pan faster than the metal can spread it out, you develop hot spots and thus get uneven heating. So in the cookware context, one way to deal with a relatively poor thermal conductor is to heat it very slowly. However, this method takes longer, it wastes energy (since a lot of it will radiate away or be taken up by air molecules and heat your kitchen–which has the potential to raise your air conditioning bills and generally make life unpleasantly hot in the kitchen), and some materials are such poor conductors that you would have to nearly turn the heat off (turn the water down to a trickle). Cast iron and carbon steel are about the lowest one can go and still get somewhat acceptably even heating for frying temperatures. If you have the time and patience, I suggest using low heat or preheating in an oven, where air currents will evenly heat the cast iron for you (at the expense of even more time, patience, and energy).
For example, see the below series of photos comparing All-Clad Stainless (2.6 mm total thickness) to Lodge Logic cast iron (~5 mm thick, but the actual thickness is hard to measure due to the bumpiness of cast iron and variation even between pieces with the same model number). I heated both on a Cooktek MC1800 induction cooker on medium heat.
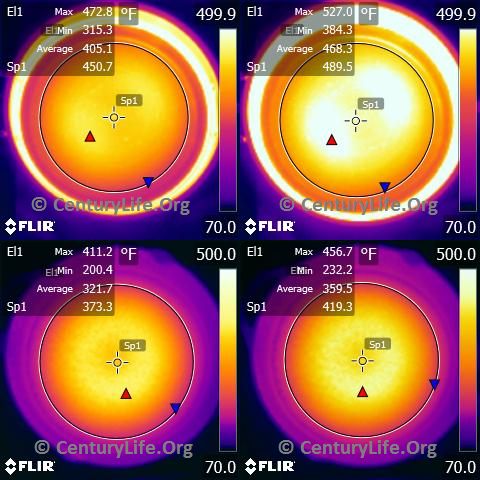
The top images are All-Clad. The bottom images are Lodge Logic Cast Iron. The images on the left are after four minutes. The images on the right are after five minutes. The circles are ~21 cm diameter (unlike my usual 20 cm for testing cookware in general, because these pans are large enough to accommodate larger circles without error from infrared reflections). As you can see, the All-Clad Stainless is about ready to saute or fry after four minutes and is reasonably even (most people tend to put food in the middle of the pan, and 158F difference is workable with some stirring). The cast iron isn’t hot enough after four minutes, and even after five minutes, there is a large difference between the hottest and coldest parts of the bottom cooking surface (~225 degrees F, or ~125 degrees C).
Now, you would think that you could simply pull up a Wikipedia article or something and be able to compare aluminum cookware with copper or cast iron cookware, but it’s not that simple. Why? Because as of 2013, a) Wikipedia is wrong, and b) in real life, cookware manufacturers use alloys rather than pure metals.
Wikipedia is wrong when it comes to thermal conductivity of certain materials. (Edit: I wrote that Wikipedia was wrong and it was, in 2013. They fixed it years later.)
Wikipedia’s entry on thermal conductivity borrows heavily from Engineering Toolbox, a site that claims nonsensical numbers sometimes; see, e.g., Engineering Toolbox’s data on pure aluminum’s thermal conductivity.1 There is no way that pure aluminum’s thermal conductivity should go from 205 W/m-K at room temperature to 250 W/m-K at 225C. It is well known that thermal and electricity conductivity in metals is highly correlated2, and all the data I’ve seen shows that aluminum does not significantly increase its electrical conductivity going from 20C to 225C.
Efunda.com (Engineering FUNDAmentals) is more credible. According to efunda.com’s data on pure aluminum (http://www.efunda.com/materials/elements/TC_Table.cfm?Element_ID=Al), thermal conductivity for aluminum barely changes for common cooking temperatures, holding pressure constant at 1 atmosphere (i.e., the air pressure that exists at sea level). For the entire range from 300 Kelvin to 500 Kelvin (80.33F to 440.33F, or 26.85C to 226.85C), pure aluminum’s thermal conductivity varies from 237 W/m-K at 80.33F (near room temperature) to W/m-K, climbing briefly to 240 W/m-K before falling back to 236 W/m-K at 440.33F. (This could be measurement error and the actual value is a constant 237 W/m-K or something like that.)
For the same temperature range (300K to 500K, or 80.33F to 440.33F), copper (of unspecified purity though likely C11000 copper since it is so common and relatively pure[2]) continuously loses thermal conductivity, dropping from 401 W/m-K to 386 W/m-K.
Cookware manufacturers use alloys, not pure elemental metals.
You might be tempted to conclude based on the above information that aluminum has roughly 60% the thermal conductivity of copper and stop there. In practice, though, nobody uses pure aluminum except as ultra-thin bonding layers that do not matter for heat conduction measurement purposes. The cookware industry uses aluminum alloys for thermal conductive layers. In marketing materials you will always find references to “aluminum” or “aluminum alloy” as the thick, thermally-conductive layer. “Aluminum” by itself is ambiguous and a way to imply that it is pure aluminum, when in fact it is aluminum alloy.
Why do cookware manufacturers use aluminum alloy for the bulk of their conductive layers, rather than pure aluminum? Answer: alloys of aluminum have superior strength and corrosion resistance relative to pure aluminum. The downside is that the strength and corrosion resistance comes at the price of lower thermal conductivity.3
SAE International designates various grades of aluminum. Grades 3003 and 3004 aluminum are the most common aluminum alloys used in cookware, going by patents and cookware specifications at company websites. These alloys are very similar and have greater corrosion resistance than other aluminum alloys that are marginally better thermal conductors. Given how people use and abuse cookware, gaining more corrosion resistance as the expense of a small amount of thermal conductivity is probably wise. In any case, 3003/3004 aluminum alloys have thermal conductivity of 162 W/m-K at room temperature.4 Like pure aluminum, we can expect them to have a more or less constant thermal conductivity rating throughout the 70-450F range commonly used in stovetop cooking.
We must do a similar analysis of what grade of copper that cookware manufacturers actually use. But as it turns out, the cookware industry uses high-purity copper5 See also Meyer Corporation’s application for copper-core patent, US20110056956 referencing their proposed use of 99.99% pure copper, which means something purer than C11000 copper (which is guaranteed to 99.9%+ pure). C10100-grade copper is 99.99%+ pure. Pure conductive metals tend to be highly thermally conductive at low temperatures, but lose a little conductivity as temperatures increase. Impurities (alloying elements) also tend to decrease thermal conductivity.], which has the kinds of copper conductivity numbers you see strewn around the web (usually around 390-400 W/m-K at room temperature).6
As a quick sanity check, we can also look at electrical conductivity numbers. For Earth-like temperatures (not near-zero or extremely hot), for metals, electrical and thermal conductivity are extremely highly correlated.7 Since electrical conductivity stays fairly flat for copper and aluminum in the 70-500F range, we can be confident that thermal conductivity is fairly flat, too.
Will the real thermal conductivity numbers please stand up?
If you are looking for real stats on thermal conductivities, I have confidence that the following numbers are more or less accurate at sea level8:
Material | Thermal Conductivity (W/m-K) at 20C | Thermal Conductivity (W/m-K) at 227C | Density (g/cc) | Specific Heat (J/g-C) | Melting Point C | Hardness Brinell |
---|---|---|---|---|---|---|
Silver (pure) | 429 | |||||
Copper (pure) | 410 | |||||
Copper C10200 | 401 | |||||
Copper C11000 | 388-394 | 373-386 | 8.92 | 0.385 | 1065-1083 | |
Aluminum (Pure) | 237 | 236 | ||||
Aluminum (3003-H14) | 159-162 | ~160 | 2.73 | 0.893 | 643-654 | 40 |
Iron (pure) | 76.2-80.2 | 7.87 | 0.44 | 1535 | 146 | |
Tin (pure) | 66.6 | 60.7 at 100C, 56.5 at 200C, 32.6 at 232-332C | 232 (but beta crystal structure only from 18-161C) | 3.9 (falls to 2.3 at 100C) | ||
Iron (Cast) | ~50-55 | |||||
Carbon Steel (0.5 to 1% carbon) | ~50-55 | |||||
Titanium (pure) | 21.9 | 0.263 | ||||
Stainless Steel (304) | 16.2 | 8 | 0.5 | 1400-1455 | 123 | |
Glass/ceramic/porcelain | Less than 2.5 |
This means that cookware-grade aluminum (3003/3004) has about 42% the thermal conductivity of C11000 copper at 440F. Or stated the other way around, C11000 copper has about 2.3 times the conductivity of 3003/3004 aluminum alloy. (At room temperature, the ratio is slightly higher.) Sounds bad for aluminum so far, right?
But recall our water pipe analogy of thermal conductivity. Two half-clogged pipes will give you the same throughput as one unclogged pipe. So instead of using 1 mm of copper, you can use 1 x 2.3 = 2.3 mm of 3003/3004 aluminum and in theory get the same heat-spreading ability.9
In practice, the thickness of other layers (stainless, tin, aluminum, etc.) and the cooktop itself (induction, coil, radiant, halogen, gas) as well as setting (watts, or Btu/h) will impact how well a particular piece of cookware spreads heat on a particular stove. Furthermore, one needs to adjust for the density of the metal.
THERMAL DIFFUSIVITY
You can think of thermal diffusivity as taking thermal conductivity and adjusting for metal density to get a measurement for how quickly temperature changes are felt. The density of cookware metals varies but not enough to change the hierarchy: if a metal has high thermal conductivity, it has high thermal diffusivity as well. For instance, copper still clobbers aluminum, which in term clobbers cast iron, which in turn clobbers stainless steel in terms of thermal diffusivity–just like with thermal conductivity.10
Material | Thermal Diffusivity (mm²/s) |
---|---|
Silver (pure, .999) | 165.63 |
Copper | 111 |
Aluminum (pure) | 84.18 |
Aluminum Alloy 3003/3004 | 60-70 |
Tin | 40 |
Cast Iron | 17 |
Stainless Steel 304A | 4.2 |
So thermal conductivity adjusted for density, or “thermal diffusivity” tells us that you need about 2 mm of aluminum to match the performance of 1 mm of copper. Objective thermal testing verifies that this is the case (De Buyer Prima Matera = 1.8 mm copper layer; Demeyere Proline = 3.7 mm aluminum layer and they heat about as evenly as each other.)
Benefits to using aluminum versus copper for the thermal conductive layer:
1. Lower weight for the same amount of thermal conductivity.
In theory, 3003/3004 aluminum gives you about 1.65 times the thermal diffusivity of copper, pound for pound. This means that for any given level of thermal conductivity, you save ~40% the weight by using aluminum instead of copper… in theory.
(The actual reduction in weight is going to be less, because the weight of the non-conductive parts of a pan/pot will not change much. For instance, stainless steel handles and interior and exterior cladding layers will weigh about the same except for a slight increase in weight for the exterior cladding, which needs to be slightly bigger to accommodate the extra volume of aluminum.)
Why does lower weight matter? First, heavy cookware can be obnoxious to move around even before you begin cooking. It may be dangerous for weaker people to move around heavy cookware, especially onto or off of high shelves. If you aren’t weak yet, you may become weaker after an accident or simple aging. Second, some people like to physically move their cookware during cooking, such as “jumping” pans (sautéing). Keep in mind that full pans weigh more than empty pans.
2. Aluminum is cheaper. Yes, you need more aluminum to match a given amount of copper, but aluminum is 3.27 times less dense than copper and costs about a third as much per pound (in 2015), so it’s still far cheaper to use 2 mm of aluminum rather than 1 mm of copper. And that’s just in raw material costs, not including the extra difficulty of shaping copper, which is harder than aluminum.
3. Aluminum is lower-maintenance than copper. Aluminum quickly oxidizes, but the thin layer that oxidizes is invisible even as it protects against corrosion. Copper is corrosion-resistant by its very nature, but it also turns crazy colors as it heats up and oxidizes, eventually developing a dull brown patina that some people think is ugly or dirty-looking. The patina won’t affect thermal performance, but it’s still a far cry from the shiny pink color of unoxidized copper, so many people resort to cleaning their copper on a regular basis to prevent too much patina from building up.
Possible benefits OR drawbacks to using aluminum versus copper for the thermal conductive layer:
1. It takes a few more millimeters of aluminum to get the same thermal conductivity as copper, so all else equal that raises your cooking surface a few millimeters in order to accommodate the extra thickness of aluminum. If your cooktop was already feeling too high or bumping up against your range hood, you might not like the extra millimeters. But it’s just another pro if your cooktop feels too low. Either way, a few millimeters is unlikely to be a dealbreaker.
2. Aluminum has much higher heat capacity than copper per gram. In fact, aluminum actually stores more heat than stainless, copper, or cast iron pound for pound–it’s just a matter of making the aluminum thick enough so that it weighs as much as stainless, copper, or cast iron. This is a blessing and a curse.
The blessing: A 2 mm layer of aluminum will soak up about 40% more heat than a 1 mm layer of copper. This is a blessing when you are doing things like searing steaks, because you want a lot of heat to be in the pan itself so that a cold steak won’t drop the temperature of the pan too much. Our hypothetical 1 mm-thick copper pan will drop in temperature faster compared to our hypothetical 2 mm-thick aluminum pan, so you may have to spend the next minute waiting for your cooktop to re-heat the copper back up to proper temperatures.
The curse: High heat capacity is bad if you want fast thermal response, especially when heating up cookware (using more energy to heat a pan to a given temperature), and arguably for cooling cookware as well–some may argue that our 2 mm-thick aluminum pan takes longer to cool down than our 1 mm-thick copper pan, thus making the copper pan better for “saving” ingredients that are about to burn. However, thermal responsiveness on the downward side is not as important as thermal responsiveness on the upward side, because if you really want to cool something down fast, you can simply take the pan off the cooktop altogether and put it on an unused burner or a heavy aluminum or copper slab.
Given the above, it is no wonder that most manufacturers have gone away from traditional copper cookware towards aluminum cookware.
There is a caveat, though, if you want cookware that conducts heat well even up the sides, especially if you want to avoid PTFEs AND want induction:
For copper, it’s easy, albeit expensive, to buy copper cookware that is 2.3 mm+ thick all the way up the sides, with a thin, nontoxic tin or stainless steel lining. (You will sometimes see “2 mm” or “2.5 mm” stainless-lined copper being advertised, but that is including a 0.2mm thick stainless steel layer, so the actual copper layer is 1.8 mm or 2.3 mm thick, respectively. Copper is toxic in large quantities and can leach into acidic foods, so it would be unsafe to not line the copper with something nontoxic like tin, silver, or stainless steel. It would be relatively safe to cook with bare copper if you knew for a fact that the food was not acidic, and some people use copper pans specifically for beating eggs as one example.) You can even get 2 mm (well, 1.8 mm + 0.2 mm) induction-compatible copper (de Buyer Prima Matera).
In contrast, for aluminum, it is very difficult to buy cookware with a thick (3.6mm+) aluminum layer that runs all the way up the sides AND is induction-compatible AND has a non-PTFE interior surface. Not impossible, though; see, for example, Berndes Signocast/Vario Click.
If you want all three, Demeyere’s Proline series is 4.8 mm thick with 3.7 mm of aluminum alloy core, but the rest of Demeyere’s cladded lineup either has a lot less aluminum (2.2 mm aluminum core for the larger saucieres; thinner than that for the smaller saucieres and woks), or is not cladded up the sides at all (the rest of the Atlantis line).
If you don’t care about the induction-compatible part, All Clad’s MC2 is made of aluminum with stainless lining. (All-Clad also used to have an anodized LTD line similar to MC2, but it appears to be discontinued except for a few pans–which are PTFE-lined anyway.) The total thickness of MC2 is roughly 3.45mm, including the stainless steel layer.
If you don’t care about induction-compatibility or PTFE lining and just want thick aluminum all the way up the sides, there are many companies out there that make relatively thick nonstick aluminum pots and pans. However, I question the economic wisdom of paying premium prices for thick aluminum cookware that has PTFE coatings that may not last. (A possible exception that is also induction compatible is Anolon Nouvelle Copper.) Furthermore, it is difficult to recycle PTFE-lined cookware, for those who are concerned about environmental effects as well.
Now, if you don’t care about even heating, it is ludicrously easy to buy All-Clad or All-Clad knockoffs that have ~1.7 mm or less of aluminum conductive layer, but such thin layers of aluminum make for somewhat uneven heating, especially on non-gas stoves, in addition to other problems such as increased risk of warping (cookware that becomes less flat over time).
As for cast iron and carbon steel, the table above implies that you need cast iron/carbon steel to be about 2.9 to 3.2 times as thick as 3003/3004 aluminum to have the same thermal conductivity. Lodge/Staub/Le Creuset are all about 4 mm thick, which equates to about 1.35 mm of aluminum and 0.5 mm of copper at searing temperatures, assuming thermal conductivity for the cast iron/carbon steel is about 50-55 W/m-K even at 440F. In other words, a cheap, nonstick 2 mm aluminum pan should easily outperform even the most expensive cast iron or carbon steel when it comes to even heating!
SPECIFIC HEAT
Thermal conductivity is not the only thermal property we care about in cookware. Specific heat also matters. We already addressed this above, though, as both a pro and a con of using aluminum instead of copper as a thermal conductive layer. “Heat capacity” is the amount of heat required to raise the temperature of a material by one degree. “Specific heat” is the ratio of the heat capacity of a material divided by that of water at 15 degrees C.
FOOTNOTES